Predicting Materials Performance in extreme Fusion Environments
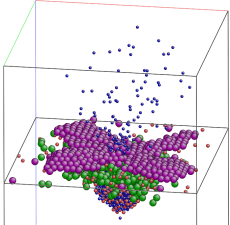
Snapshot from a molecular dynamics simulation showing evolution of a helium bubble below a tungsten surface just before the bubble ruptures. Red spheres are vacant sites, green are displaced tungsten atoms, purple are surface tungsten adatoms, blue spheres are helium atoms.
What it Matters: Harnessing nuclear fusion as a commercially viable energy technology requires advances not only in fusion physics but also in materials science. This is because of the enormous heat, radiation, gas formation, and corrosive conditions experienced by plasma-facing metals in fusion reactors. This project seeks to develop a predictive capability to discover mechanisms of materials degradation in extreme fusion environments with a view towards providing practical engineering solutions to enable fusion energy.
Key Challenges: Initial efforts are in modeling the mechanism of radiation damage in tungsten, to extend the lifetime of "divertor" devices that will be used for removal of material from the plasma during operation of fusion reactors such as ITER. This requires development of detailed physics models and computational strategies over a wide range of length and time scales, as well as integrating extensive physical processes across the plasma - surface - bulk materials boundaries. This project simultaneously attacks these problems from a “bottom-up” atomistic-based approach and “top-down” continuum perspective that focuses on kinetic models of species reactions and diffusion.
Accomplishments: Molecular Dynamics (MD) simulations performed using the Large-scale Atomic/Molecular Massively Parallel Simulator (LAMMPS) software have revealed sub-surface mechanisms likely involved in the initial formation of nanometer-sized pitting in tungsten exposed to low-energy helium plasmas. This is a key result because tungsten is a leading candidate as a divertor material due to its many favorable characteristics. The simulations demonstrate several atomic-scale, sub-surface phenomena that lead to initial formation of nanoscale tungsten ‘fuzz’ observed in laboratory experiments. Helium from the plasma becomes implanted within the metal but helium atoms are relatively mobile and have a strong driving force to cluster, this simulation found. Repeated cycles of helium absorption and metal lattice defect formation causes the clusters to form bubbles that burst. This, and movement of individual "adatoms" of tunsten to the surface, cause the surface roughening. Thus, MD simulations such as these, which have been performed using nearly 25 million atoms, have accurately captured the key mechanisms associated with surface erosion.
Investigators: B. Wirth, Karl Hammond, Faiza Sefta (University of Tennessee)
More Information: See, for example, "Tungsten surface evolution by helium bubble nucleation, growth and rupture," Nucl. Fusion 53 (2013) 073015; doi: 10.1088/0029-5515/53/7/073015