Exploring the High-Pressure Materials Genome
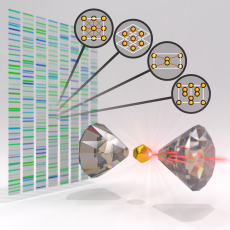
A new framework for predicting materials behavior at high pressure combines computational approximations with extant materials data to provide a tool for discovering new materials and exploring their properties under nonambient pressure conditions
Scientific Achievement
Researchers at Northwestern University developed a novel computational framework that runs on NERSC’s Cori supercomputer to rapidly explore apparent paradox of material phases that exist in nature that should be unstable according to calculations that assume idealized conditions of zero pressure and zero temperature. Using this method, the stability of these material phases is better understood, and the team has identified several new intermetallic high-pressure phases that can be realized with existing experimental techniques.
Significance and Impact
We are heading towards an age of materials design where new materials are discovered at an increasing rate and functional materials are designed with tailored properties to tackle global environmental challenges. Theory, computational modeling, and data-driven approaches are rapidly evolving and actively assist in this endeavor. Materials discovery efforts using computed materials data have shown great success in predicting new materials.
Unfortunately, most currently available databases only contain materials properties at idealized conditions of zero pressure and zero temperature, thereby limiting their application to thermodynamic ground states at ambient conditions. However, it is well known that many relevant materials for energy applications are in fact not thermodynamic ambient ground states, but are metastable. The significance of metastable phases in numerous practical applications calls for advanced techniques to sample the phase stability of a large number of materials at non-ambient conditions.
A large-scale analysis of experimentally reported compounds using the developed linear approximation of the enthalpy (LAE) framework shows that around 60% of all observed ambient-metastable phases are actually thermodynamically stable at some finite pressure. Most ambient-metastable phases in nature can thus be understood to be remnants of high-pressure conditions where they form as the stable ground states. Such extreme conditions could even occur during synthesis at atmospheric pressures, for example as local pressure fluctuations or chemical pressure. In its current form, the presented framework will directly impact explorative efforts in geological and planetary science, e.g., determining phase compositions of planetary interiors. Further, it can be readily expanded into a comprehensive phase stability framework by including other thermodynamic variables such as temperature, electric potential, and size of nanoparticles. Overall, this work paves the route towards robust exploration of the entire materials genome based on emerging materials big data, leading to accelerated materials discovery at non-ambient conditions.
Research Details
The researchers used their novel techniques in conjunction with calculated materials properties in the Open Quantum Materials Database (OQMD) to explain why some compounds remain relatively stable in the environment. Further, more than 3,500 new compounds that are stable at finite pressures were predicted. The investigation used 800,000 hours of compute time on Cori.
Of all the phases observed in nature, nearly 50% are calculated to be not thermodynamically stable at T=0 K. This apparent paradox is partly resolved by using the LAE method to analyze the phase stability of all known compounds at non-ambient pressures. A large fraction of the ambient-metastable phases (up to 60%) are found to be thermodynamically stable at some finite pressure. In other words, more than half of all ambient-metastable phases are possibly remnants of high-pressure conditions in which they formed as thermodynamic ground states.
Finally, the researchers show that their model can be used to discover completely new materials by combining the LAE framework with a sophisticated crystal structure prediction method. Focusing on a unique subset of around 2000 binary systems which are immiscible at ambient pressures, the stability of hypothetical phases was analyzed and used to screen for binary systems with potential high-pressure materials. Ten of these candidate phase spaces were studied in detail using the minima hopping crystal structure prediction method by performing ab initio structural searches. In nine out of the ten ambient-immiscible systems, the combination of the LAE with MHM correctly predicted that new compounds can be formed at sufficiently high pressures.
Related Links
Amsler, Hegde, Jacobsen, and Wolverton, Phys. Rev. X 8, 041021 (2018),
doi: 10.1103/PhysRevX.8.041021
About NERSC and Berkeley Lab
The National Energy Research Scientific Computing Center (NERSC) is a U.S. Department of Energy Office of Science User Facility that serves as the primary high performance computing center for scientific research sponsored by the Office of Science. Located at Lawrence Berkeley National Laboratory, NERSC serves almost 10,000 scientists at national laboratories and universities researching a wide range of problems in climate, fusion energy, materials science, physics, chemistry, computational biology, and other disciplines. Berkeley Lab is a DOE national laboratory located in Berkeley, California. It conducts unclassified scientific research and is managed by the University of California for the U.S. Department of Energy. »Learn more about computing sciences at Berkeley Lab.